The ancient Universe is weird and secretive. Scientists have made laudable progress in uncovering more and more information on how the Universe began and what conditions were like all those billions of years ago. Powerful infrared telescopes, especially the ground-breaking James Webb Space Telescope, have let astronomers study the ancient light from the early Universe and remove some of the secrecy.
One of the mysteries astronomers want to untangle concerns star formation. Has it changed much since the Universe’s early days?
The Universe has evolved a lot since the first stars formed, and astrophysicists want to know if stars form differently now than they did billions of years ago. There are a couple of ways to study the ancient Universe’s star formation. One is to use the JWST and its ability to effectively ‘look back in time.’ But observing time on that telescope is in extremely high demand. Another way to do it is to study a place in the modern Universe that mimics the ancient Universe.
That’s what a group of Japanese researchers did.
The primary difference between stars that formed billions of years ago and stars that form now concerns the material available during the process. Not only the amount of material but also how enriched it is. This is referred to as stellar metallicity. Many Universe Today readers know that in astronomy, elements heavier than hydrogen and helium are called metals. These metals only come from one place: the stars themselves.
Stars create elements heavier than hydrogen and helium through nucleosynthesis. The intense conditions inside massive stars create elements like oxygen, iron, carbon, and everything in the periodic table beneath H and He. When these stars explode as supernovae or shed their outer layers as red giants, these elements are cast out into space, available for the next round of star formation.
We live in a Universe where countless stars have lived and died, synthesized heavy elements and spread them out into space. Consequently, stars in the modern Universe are formed from a variety of elements. But the Universe’s first stars didn’t have metals available to them and consisted of only hydrogen and helium. (As a side note, any planets that formed around these original stars couldn’t have been anything like Earth. Without previous generations of stars and the heavier elements they synthesized, rocky planets wouldn’t exist, and neither would we.)
The question astronomers want to answer is do stars form differently in a low-metallicity environment, a.k.a. the ancient Universe, versus the modern, metal-rich Universe. To find out, a team of researchers located a star-forming region in the Milky Way exhibiting low metallicity that’s similar to the primitive Universe, compared to the higher metallicity in the contemporary Universe.
The region is called Sh 2-209 (hereafter referred to as S209.)
The research was led by Chikako Yasui, an Assistant Professor at the National Astronomical Observatory of Japan. The team used the Subaru Telescope in Hawaii to study S209, and they presented their results in a paper titled “Mass Function of a Young Cluster in a Low-metallicity Environment. Sh 2-209” published in The Astrophysical Journal.

We can quickly recap the broad strokes of the star formation process as science understands it.
Things begin in a massive structure in space called a giant molecular cloud. These clouds contain everything available to a nascent star. The clouds are dominated by hydrogen, the most abundant element in the Universe by far, and also the simplest. They’re called molecular clouds because individual hydrogen atoms don’t want to be alone. They love to hold hands, and so they bond together as a molecule containing two atoms of hydrogen.
These clouds are not perfectly uniform in their structure. There are irregularities, regions where the gas is more or less dense, and exhibits different temperatures, motions, and velocities. Eventually, small knots or clumps of gas form in these clouds, and over time their gravity draws more gas into them. Eventually, enough material is drawn in to form a protostar, which gives off some energy but hasn’t begun fusion yet. Only when enough mass has gathered to drive the core pressure and temperature to extremes does fusion begin. Then we have a star, beginning its long journey along the main sequence.
But how do differing levels of metallicity affect the stars that result from this detailed process? Astrophysicists know a couple of things.
Higher metallicity can result in stars with lower temperatures. Stars with higher metallicities can be cooler while they’re on the main sequence and on the giant branch. They can also appear redder. None of this is particularly surprising. Even though metals, meaning elements heavier than hydrogen and helium, make up only about 2% of the Universe’s baryons by mass, they have a powerful effect on heating and cooling during star formation. Metallicity is one of the most critical factors in star formation.
But when it comes to stars, mass is king. A star’s future is determined by its mass. Huge stars, many times more massive than our Sun, burn hot and don’t last long. These can end as supernovae. Stars much less massive than our Sun are called low-mass stars, and they vastly outnumber high-mass stars. These stars, including the plentiful red dwarf stars, can live for trillions of years.
Stars seldom form in isolation. Typically, they form in clusters, and in these clusters, the masses of individual stars are similar. Would stars forming in the early low-metallicity Universe maintain this similarity in masses?
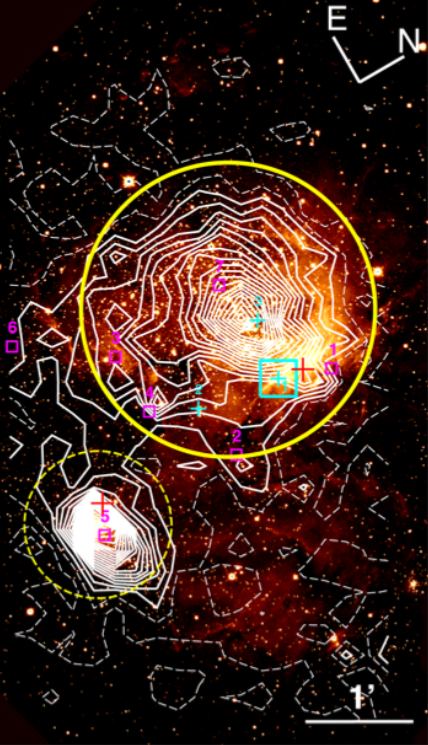
S209 can help answer that question because it has another thing going for it other than its low metallicity. It’s close, only about 2.5 parsecs (8.1 light-years) away. “This is close enough to enable us to resolve cluster members clearly (~1000 au separation) down to a mass-detection limit of ~ 0.1 solar masses,” the paper says, “and we have identified two star-forming clusters in S209, with individual cluster scales ~1 pc.”
Detecting the mass of the stars in S209 down to 0.1 solar masses is obviously important. It means that observations have less room for error, making the study’s results more robust.
S209 holds two separate star clusters. One is large, and one is small, and the larger one contains up to 1500 stars. This work marks the first time that astronomers have identified a cluster with this many identifiable members in the Milky Way’s outer regions. It’s critical because other, similar regions contain only about 100 stars, a sample too small to draw reliable conclusions from. Not only that, but the Subaru Telescope’s power identified stars as small as 1/10 the Sun’s mass, up to stars 20 times the Sun’s mass.
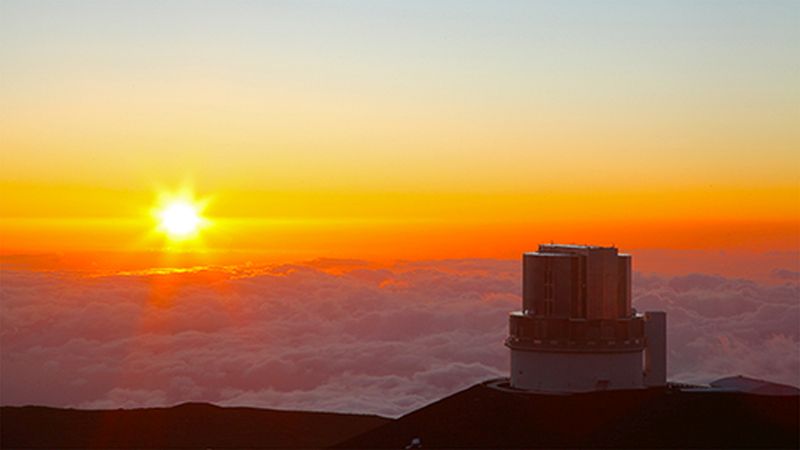
What’s the significance of this? It all comes down to what’s called the Initial Mass Function (IMF), and its utility in understanding stars, solar systems, and even galaxies.
The IMF describes the mass distribution of a population of stars that formed together. So it not only describes how stars form and evolve, but it also helps describe how galaxies form and evolve. The IMF can help predict how many different types of stars and stellar objects will be contained in a given region, a critical part of understanding the Universe.
When coupled together, the IMF and the stellar metallicity help dictate how stars will form in a given cluster. As stars form, they regulate their own mass through self-radiative feedback. The effect of self-radiative feedback is more pronounced in a low-metallicity environment, so these environments should produce more high-mass stars.
The results of this study bear that out, and the research turned up some interesting results. S209 contains a few more stars that are more massive than other star-forming regions in the neighbourhood, but only slightly more. S209 also has slightly more stars that are less massive than the Sun.
“The outer part of the Milky Way is known to have properties similar to those of the early Universe,” said Yasui. “Our results suggest that although a relatively large number of massive stars formed in the early Universe, the number is not dramatically different from that of typical star clusters in the present day.”
So is the ancient Universe weird and secretive like we said at the opening of this article? Almost certainly, especially when it comes to things like dark energy and dark matter, modern cosmology’s two unassailable monoliths.
But these results show that the weirdness didn’t pervade everything. Stars are the Universe’s basic building blocks, and this research indicates that they formed much the same way back then as they do now.
The post Star Factories Haven’t Changed Much Over the Entire Age of the Universe appeared first on Universe Today.
No comments:
Post a Comment