The effects of Climate Change on Earth’s living systems have led to a shift in biological studies, with attention now being focused on the boundaries within which life can survive. Studying life forms that can thrive in extreme environments (extremophiles) is also fundamental to predicting if humans can live and work in space for extended periods. Last, but not least, these studies help inform astrobiological studies, allowing scientists to predict where (and in what form) life could exist in the Universe.
In a recent study, a team of Italian researchers used brine shrimp (Artemia franciscana) in the earliest stage of development (nauplii) and subjected them to Mars-like pressure conditions. Their results indicate that while the nauplii experienced physiological changes, their development remained largely unchanged. This not only demonstrates that extremophiles show great adaptability and can survive in Mars-like conditions. It also indicates that similar life forms could be found elsewhere in the Universe, representing new opportunities for astrobiological research.
Maria Teresa Muscari Tomajoli, an Astrobiology PhD Candidate at the Parthenope University of Naples, led the study. She was joined by Paola Di Donato, a Professor of Organic and Biological Chemistry at Parthenope. They were joined by researchers from the Federico II University, the INAF-Institute of Space Astrophysics and Planetology (INAF-ISAP), the INAF-Osservatorio Astronomico di Capodimonte, and the Italian Institute for Nuclear Physics (INFN). The paper that details their findings was part of a special volume titled Comparative Biochemistry and Physiology A: Molecular & Integrative Physiology.

On Earth, extremophiles belong to all three domains of life (Archaea, Bacteria, and Eukarya). They are characterized by their ability to withstand pressure, acidity, temperatures, and other conditions that would be fatal to other organisms. After Earth, Mars is considered the most habitable planet after Earth in the Solar System, hence why most of humanity’s astrobiology efforts are focused there. In addition to the low atmospheric pressure (1/100th of Earth’s at sea level), the surface is subject to extreme temperature variations and is contaminated by perchlorites and toxic metals.
Scientists speculate that if life exists on Mars today, it will likely take the form of microbes living in high-salinity briny patches beneath the surface. As Tomajoli told Universe Today via email, this makes extremophiles (like Artemia franciscana) ideal test subjects for predicting what life is like in similar planetary environments:
“The definition of life is crucial, especially when searching for traces of it on other planetary bodies (e.g., Mars), where life might not exist as we traditionally imagine it. Artemia cysts present an interesting case: in their dormant state, they cannot be classified as living but rather as potential life. Studying organisms with such characteristics helps broaden the perspective in astrobiological research.”
In particular, extremophiles present opportunities for researching species adaptation, which has become a major focus of scientific research due to anthropogenic Climate Change. Worldwide, rising carbon emissions and increasing temperatures are leading to changes in weather patterns, increased ocean acidity, drought, wildfires, and the loss of habitats. As a result, countless marine and terrestrial species are forced to adapt to conditions that are becoming more extreme.

“In the context of climate change, life conditions are shifting toward extreme boundaries, making survival more challenging for many organisms,” Tomajoli added. “Extremophiles, which thrive in Earth’s most remote environments, are valuable models for understanding metabolic adaptations. Their apparent simplicity is, in fact, an advantage, allowing them to adapt better than more complex organisms to extreme environmental constraints.”
Tomajoli and her colleagues chose Artemia franciscana for their study, a species of brine shrimp known to thrive in high-salinity environments. The eggs they produce, known as cysts, are dormant and can be stored indefinitely, making them extremely useful for aquaculture and scientific research. As Tomajoli indicated, they have also been used in previous space missions, including the Biostack experiment on the Apollo 16 and 17 missions and the ESA’s EXPOSE platform mounted on the International Space Station’s (ISS) exterior.
These experiments all tested the resilience of certain life forms and their progeny to cosmic rays. However, as Tomajoli added, no further studies have been conducted regarding the physiological adaptations of Artemia franciscana, and there is currently no scientific literature available on the topic:
“In particular, Artemia brine shrimps are considered halophiles (literally “salt-loving” organisms) and thrive in environments that can be considered Mars analogs (or laboratories for Mars studies) such as temporary lakes that undergo frequent evaporation, prompting Artemia to produce cryptobiotic cysts. Additionally, Artemia is an easily cultivable model, making it suitable for biological and astrobiological experiments. A recent article by Kayatsha et al., 2024 also showed that Artemia franciscana was among all the microinvertebrates that were tested, the more resistant one to perchlorates salts present in the regolith of simulated martian soil.”
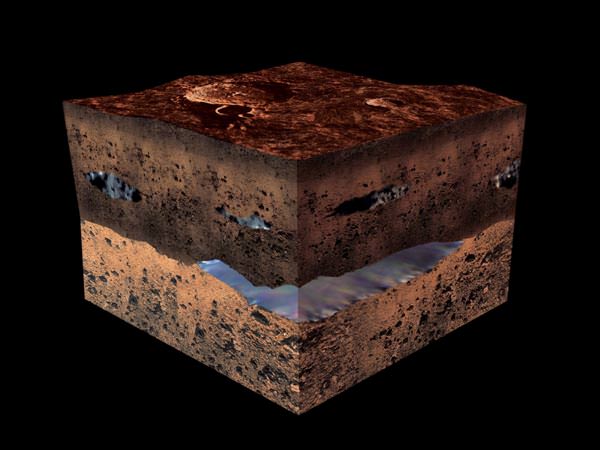
For their experiment, Tomajoli and her colleagues placed dormant cysts in Mars-like pressure conditions. Once they hatched into nauplii, the team analyzed their aerobic and anaerobic metabolism, mitochondrial function, and oxidative stress. As indicated in their paper, brine shrimp born in Martian pressure conditions managed to adapt quite well. They further share how these results could lead to further studies to evaluate the metabolic adaptations of the cysts to longer exposure times, combinations of different Mars-like conditions, or studies of the adaptations of the nauplii in other stages of development:
“Artemia franciscana showed an exciting potential for physiological adaptations, enabling organisms to cope with the environmental challenges they encounter in space… Nauplii’s cells appear to activate responses to avoid mitochondrial dysfunction and continue their growth processes. These adaptation mechanisms highlight Artemia franciscana’s resilience and ability to thrive in hostile environmental conditions. The results reported in this study further support the potential use of Artemia franciscana for astrobiological purposes, highlighting the animals’ metabolic and redox state changes as a response to adaptation to an extreme condition mimicking the space.”
The implications of this research are far-reaching, embracing astrobiology, human space exploration, and mitigating the effects of Climate Change. Not only could it help point the way toward potential life on Mars, in the interior oceans of icy bodies, and other extreme environments. It could also inform future missions to Mars and other deep-space destinations, where astronauts will need to rely on closed-loop bioregenerative life support systems (BLSS), grow their own food, and conduct research into the effects of exposure to lower gravity, elevated radiation, and other harsh conditions.
At home, the study of extremophiles and adaptation mechanisms could provide insight into climate resilience and adaptation, consistent with the goals outlined in the Sixth Assessment Report (AR6) by the Intergovernmental Panel on Climate Change (IPCC). As they summarize in their paper:
“Understanding the mechanisms of Artemia franciscana adaptations to space-simulated conditions could provide new insights into the study of the limits of life, as well as contribute to the search for biosignatures—traces of past life on other planetary bodies. Additionally, it could offer a viable solution for the long-term survival of human space missions, helping establish self-sustaining populations in confined environments. Artemia could serve as a renewable food source for astronauts, given its richness in essential nutrients, including proteins, lipids, and vitamins.”
Tomajoli and her colleagues have also conducted simulations with a full Mars-like atmosphere for longer periods of time. The paper describing this experiment will be released soon. In the meantime, the search for life on Mars and beyond continues. Knowing it can exist out there and under what conditions will help narrow that search and encourage us to keep investigating.
Further Reading: Science Direct
The post How Brine Shrimp Adapted to Mars-like Conditions appeared first on Universe Today.