When a prominent star in the night sky suddenly dims, it generates a lot of interest. That’s what happened with the red supergiant star Betelgeuse between November 2019 and May 2020. Betelgeuse will eventually explode as a supernova. Was the dimming a signal that the explosion was imminent?
No, and new research helps explain why.
Headline writers couldn’t resist the supernova angle, even though that explanation was never very likely. Eventually, it became clear that ejected dust from the star caused the dimming. New research based on observations before, during, and after the Great Dimming Event (GDE) supports the idea that dust from the star itself caused Betelgeuse’s drop in brightness.
A research letter titled “Images of Betelgeuse with VLTI/MATISSE across the Great Dimming” presents the infrared observations of Betelgeuse. The observations capture the star before, during, and after the GDE. The lead author is Julien Drevon, from the Université Côte d’Azur, France, and the European Southern University.
“To better understand the dimming event, we used mid-infrared long-baseline spectro-interferometric measurements of Betelgeuse taken with the VLTI/MATISSE instrument before (Dec. 2018), during (Feb. 2020) and after (Dec. 2020) the GDE,” the research letter states. In particular, their observations focus on silicon monoxide (SiO.)
The authors of the new research outline three steps in the process that created the GDE.
Step One
The GDE started with shocks deep inside Betelgeuse. They generated a convective outflow of plasma that brought material to the star’s surface. Researchers detected a strong shock in February 2018 and a weaker one in January 2019. The second, weaker shock boosted the effect of the stronger shock that preceded it, generating a progressive plasma flow at the surface of Betelgeuse’s photosphere.
Step Two
The plasma flowing to the photosphere’s surface created a hot spot. Hubble UV observations of Betelgeuse revealed the presence of a luminous, hot, dense structure in the star’s southern hemisphere, between the photosphere and the chromosphere.
Step Three
Stellar material detaches from the photosphere and forms a gas cloud above Betelgeuse’s surface. A colder region forms under this cloud as a dark spot. Since it’s cooler, dust is allowed to condense above this region and in the part of the cloud above it. That dust is what blocked some of Betelgeuse’s luminosity, causing the GDE.
Previous research revealed this three-step process behind the GDE. The authors of the new research article set out to observe Betelgeuse’s close circumstellar environment to probe and monitor its geometry. In the wavelength range they worked in, SiO spectral features are prominent, and they’re used to understand what happened with the red supergiant. In astronomy, SiO is used as a tracer for shocked gas in stellar outflows since it persists at high temperatures.
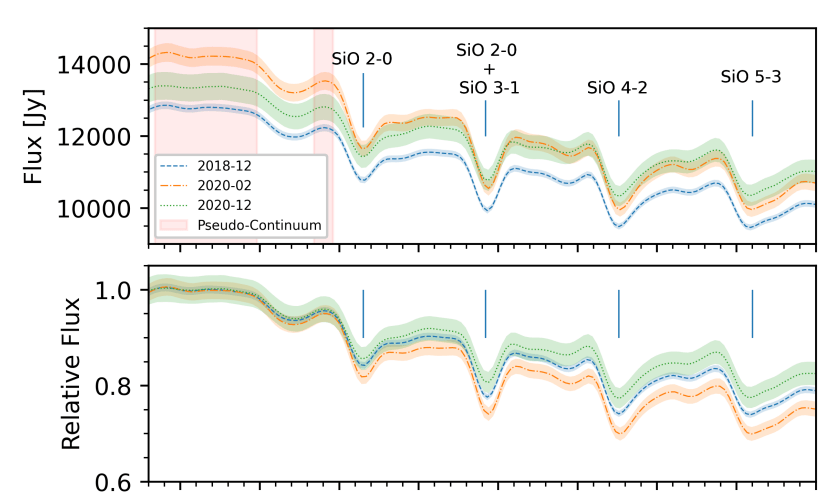
In their article, the authors focus on the SiO (2-0) band and what it signifies. They note how the band’s intensity contrast increases by 14% during the GDE. “Therefore, it seems that during the GDE, we observe brighter structures in the line of sight,” they explain.
Next, they note a 50% decrease in intensity contrast in December 2020. What does it mean?
“The SiO (2–0) opacity depth map shows, therefore, strong temporal variations within 2 years, indicative of vigorous changes in the star’s environment in this time span,” they write.
Their observations also suggest “the presence of an infrared excess in the pseudo continuum during the GDE, which has been interpreted as new hot dust formed,” Drevon and his colleagues write.

It seems like the Great Dimming is no longer the mystery it once was. It also shows that Occam’s Razor is alive and well: “The explanation that requires the fewest assumptions is usually correct.”
The supernova proposal was fun for a while, and one day, Betelgeuse will explode as a supernova. But before it ever does, there are likely going to be several more episodes of dimming. For now, the authors say that the star is returning to normal.
“The Dec. 2020 observations suggest that Betelgeuse seems to be returning to a gas and surface environment similar to the one observed in Dec. 2018,” they write, “but with smoother structures, maybe
due to the unusual amount of dust recently formed during the GDE in the line of sight.”
Case closed?
The post Betelgeuse. Before, During and After the Great Dimming appeared first on Universe Today.