The fields of astronomy and astrophysics are poised for a revolution in the coming years. Thanks to next-generation observatories like the James Webb Space Telescope (JWST), scientists will finally be able to witness the formation of the first stars and galaxies in the Universe. In effect, they will be able to pierce the veil of the Cosmic Dark Ages, which lasted from roughly 370,000 years to 1 billion years after the Big Bang.
During this period, the Universe was filled with clouds of neutral hydrogen and decoupled photons that were not visible to astronomers. In anticipation of what astronomers will see, researchers from the Harvard & Smithsonian Center for Astrophysics (CfA), the Massachusetts Institute of Technology (MIT), and the Max Planck Institute for Astrophysics (MPIA) created a new simulation suite called Thesan that simulates the earliest period of galaxy formation.
The creation of the Thesan suite and the results the team obtained are described in a series of three papers that were recently accepted to the Monthly Notices of the Royal Astronomical Society. The simulations were created using the SuperMUC-NG supercomputer located at the Leibniz Supercomputing Centre of the Bavarian Academy of Sciences. The process took over 30 million CPU hours and would have required more than 3,500 years to complete on a conventional computer.
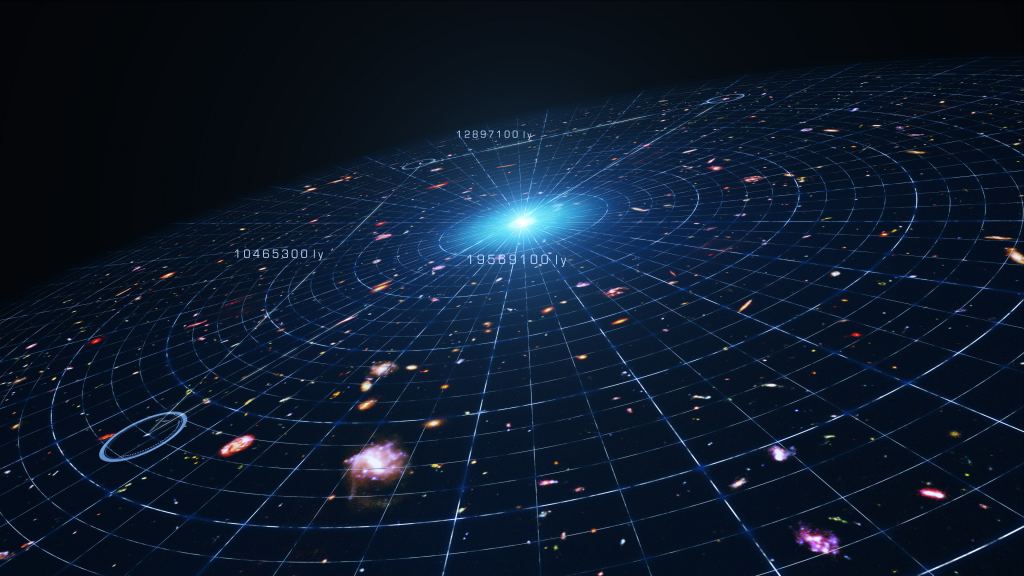
The simulations cover the Epoch of Reionization (ca. 13 billion years ago). In this cosmic period, neutral hydrogen atoms were ionized to form positive hydrogen atoms, allowing light to spread throughout the Universe. Simulating this period was no easy task, as it involved recreating some immensely complicated and chaotic interactions between gravity, gas, radiation, and more. It also meant capturing physics down to scales a million times smaller than the simulated regions.
The team accomplished this by combining realistic models of galaxy formation and cosmic dust with a new algorithm that tracks how light interacts with gas. Through this, they were able to resolve interactions in the early Universe in unprecedented detail and over the largest volume of any previous simulation. As Rahul Kannan, an astrophysicist at the CfA and the lead author of the first paper in the series, explained:
“Most astronomers don’t have labs to conduct experiments in. The scales of space and time are too large, so the only way we can do experiments is on computers. We are able to take basic physics equations and governing theoretical models to simulate what happened in the early universe.”
“A lot of telescopes coming online, like the JWST, are specifically designed to study this epoch. That’s where our simulations come in; they are going to help us interpret real observations of this period and understand what we’re seeing.”

With this new simulation suite, researchers can simulate a piece of our Universe spanning 300 million light-years in diameter. They can then run it forward in time to track and visualize the formation of the first galaxies in this space, beginning around 400,000 years after the Big Bang, and watch how they evolved during the first billion years. When the team ran the simulations, they found that the transition from complete darkness to light was gradual.
Said study co-author Aaron Smith, a NASA Einstein Fellow in MIT’s Kavli Institute for Astrophysics and Space Research:
“It’s a bit like water in ice cube trays; when you put it in the freezer, it does take time, but after a while it starts to freeze on the edges and then slowly creeps in. This was the same situation in the early Universe — it was a neutral, dark cosmos that became bright and ionized as light began to emerge from the first galaxies.”
The simulations were created to prepare for observations from next-generation telescopes like James Webb, Nancy Grace Roman, and Origins space telescopes. Along with ground-based telescopes like the Extremely Large Telescope (ELT) and the Giant Magellan Telescope (GMT), these observatories will be able to see deeper into space and (hence) farther back in time than their predecessors. In fact, it is estimated that James Webb will be able to see the Universe as it was 13.5 billion years ago.
Right now, the record for the most distant single object ever seen goes to Earendel, a star located about 12.9 billion light-years from Earth. In terms of galaxies, that record goes to GN-z11, located about 13.39 billion light-years from Earth in the constellation Ursa Major. What’s especially exciting is that the astronomical community won’t have to wait long for real telescope observations and data to be compared to Thesan simulations.
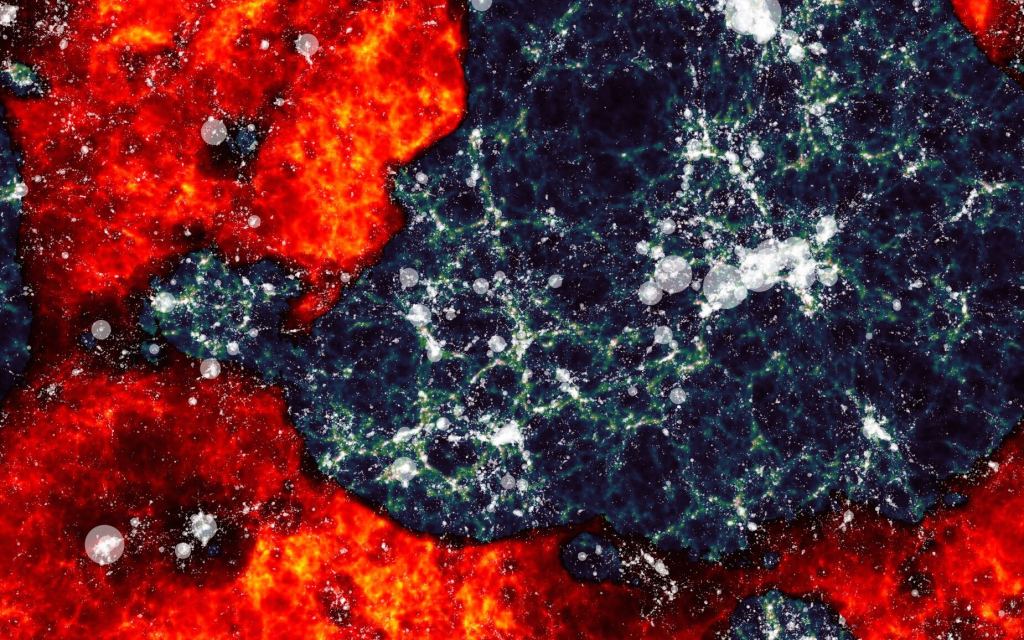
“And that’s the interesting part,” said co-author Mark Vogelsberger, an associate professor of physics at MIT. “Either our Thesan simulations and model will agree with what JWST finds, which would confirm our picture of the universe, or there will be a significant disagreement showing that our understanding of the early universe is wrong.”
However, the team won’t know how their model stacks up against the real thing until the first observations become available. Once they do, they will attempt to match various aspects of their model to the observations, including the properties of early galaxies and the absorption and escape of light in the early Universe. From this, we will finally know how and when the Dark Ages were dispelled.
“We have developed simulations based on what we know,” Kannan says. “But while the scientific community has learned a lot in recent years, there is still quite a bit of uncertainty, especially in these early times when the universe was very young.”
For generations, astronomers have waited for the day when it would be possible to view the earliest periods of the Universe and see how it all began. When paired with observations of how the cosmos has evolved since scientists will finally be able to address some of the deepest mysteries of the cosmos. To know that such a day is right around the corner is nothing short of exciting!
And be sure to check out this 3D view of the Thesan simulation. Check out more simulations on their Youtube page!
Further Reading: Harvard-Smithsonian Center for Astrophysics
The post New Simulation Recreates an Early Time in the Universe That Still Hasn't Been Seen Directly appeared first on Universe Today.